YK11: The Complete Guide
*DISCLAIMER: Selective Androgen Receptor Modulators (SARMs) are not approved by the FDA for any currently acceptable medicinal/therapeutic purpose. Therefore, this article does not serve as medical advice and is not to be intended to be used to diagnose, treat, cure or prevent any disease. SARMS are to be used for research purposes only and not intended for human consumption.*
What is YK11?
YK11 is a very unique SARM, which also makes it a little bit confusing to understand. On the one hand, it’s an anabolic-androgenic steroid (AAS). On the other hand, it’s also considered a selective androgen receptor modulator (SARM). So why is it that YK11 can be considered to be both an AAS and a SARM?
The reasoning behind YK11’s AAS classification is due to its chemical structure. Steroid hormones (e.g. progesterone, testosterone, estrogen,etc.) share similar foundations when it comes to their structural backbones. All the different types of AAS contain only minor variations within their molecular structures relative to testosterone, of which all AAS derivatives are essentially based off of.

But YK11 acts as a
partial agonist of the
androgen receptor (AR), which is why it’s also considered to be a SARM [
1]. How exactly does this make it a SARM?
It first helps to understand what exactly an AR
agonist is in the first place. Androgens act as primary activators (agonists) of the AR; the most prominent of which are testosterone and its potent derivative dihydrotestosterone (DHT). When these androgens bind to the AR, it exerts a multitude of effects; many of which are important for male development. This includes the synthesis of sperm (spermatogenesis) and the growth/maintenance of skeletal muscle tissue [
2].
Circling back to YK11’s role as a partial agonist, the “partial” aspect of its agonistic role speaks to its ability to bind selectively to the AR, hence the “selective” part of selective androgen receptor modulator. This is of course relative to the agonist DHT, which is often to blame for androgens’ undesirable effects/outcomes such as male-pattern baldness, acne, and even prostate cancer [
3]. This explains the motivation behind why researchers are interested in examining YK11’s potential use as a safer alternative to androgen therapy. But while research has suggested that YK11 has a modest ability to selectively activate the AR, it still does not match the selectivity of other SARMs.
The lack of regulation and overall “looseness” of the term “SARM” may be to blame here, as there is no minimum amount of selectivity, per se, definitively separating a SARM from an AAS. Hopefully as more research is conducted, this blurred line becomes clearer.
YK11 is a Potent Myostatin Inhibitor
YK11 has stirred up massive amounts of attention in the fitness and bodybuilding communities primarily due to its potential ability to inhibit
myostatin; a type of protein that is expressed in skeletal muscle and acts to limit overall muscle fiber growth.
Myostatin is often overexpressed in muscle-wasting conditions, such as HIV, AIDS, and cancer. It’s been shown that the overexpression of myostatin in mice induced patterns of muscle-wasting comparable to the aforementioned conditions observed in humans [
4].
Myostatin was originally hypothesized to have existed as far back as the 1960s, coming to fruition in 1997, when a group of researchers conducted a study on mice using a
gene knockout model. In research, a gene knockout model is simply when researchers remove one specific gene from an animal and compare it to an animal that still has the gene intact (control) and observe the differences.
In this specific instance, the researchers removed the myostatin gene from a group of mice and observed dramatic increases in skeletal muscle mass; with the muscles of the mice lacking myostatin weighing twice as much as the mice in the control group on average [
5].
Interestingly, these increases in muscle mass occurred as a result of both increases in the number of muscle fibers (hyperplasia) as well as increases in the total fiber size (hypertrophy). This could potentially mean that YK11 creates an additional pathway (therefore an additional opportunity) for muscle growth that other SARMs and AAS do not. However, this concept has not been officially explored.
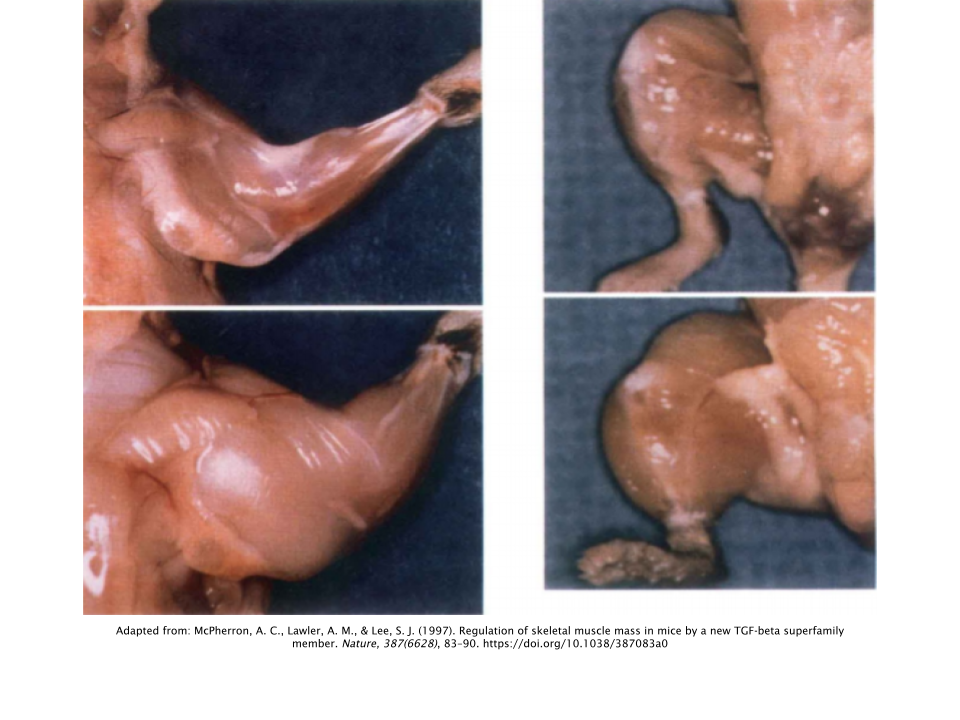
The same group of researchers had also examined this phenomenon in the Belgain Blue bull, which has a mutation in the myostatin gene that causes both greater levels of muscle accretion as well as decreased levels of adipose (fat) deposition, which results in this cattle producing very lean cuts of meat [
6]. At birth, a Belgain Blue calf is approximately twice the weight of a normal calf [
7].
Follistatin
Follistatin can be thought of as the polar opposite of myostatin; acting as its primary antagonist and inhibiting its muscle-limiting actions throughout the body. Theoretically, increased amounts of follistatin equals less circulating levels of myostatin. Thus, leading to increased rates of muscle growth.
This theory tends to be supported, with a study conducted in follistatin knockout mice (mice that lacked the follistatin gene) showing reduced amounts of muscle mass at birth, with the lack of follistatin inevitably leading to the overactive expression of myostatin [
8].

YK11’s ability to selectively bind to the AR while simultaneously promoting follistatin expression is what makes this compound so attractive to recreational users. This mechanism of action is markedly different from that of other SARMs, which further explains its double classification as both an AAS and a SARM.
YK11’s unique capability to inhibit myostatin would make for a great addition to an already existing AAS/SARM regimen, as any other compound in an already existing stack would not possess such an ability.
Recreational Use/Anecdotal Reports
Recreational users often report both notable muscle-building and muscle-hardening effects. It’s difficult to provide the mechanistic reasoning behind YK11’s effect on the latter due to a lack of human trials on YK11’s anabolic effects from a sports performance/physique enhancement context.
This muscle-hardening effect may be attributed to its relationship with DHT, as DHT-derived steroids like trenbolone and stanozolol (Winstrol™) are oftentimes used to exert this hardening effect. This would make sense, as anecdotal reports have been consistent with what would typically be observed during a cycle of DHT-derived AAS. Nobody expects to pack on
massive amounts of muscle mass with steroids such as trenbolone and stanozolol. Most will use these types of AAS during a cutting cycle since the DHT-derived AAS don’t have a propensity to cause water retention unlike other AAS. Provided this information,
YK11 would definitely be better suited during one’s cutting cycle.
Side Effects
Unfortunately, the lack of human data makes it extremely difficult to assess the potential for side effects to occur while taking YK11. However, in keeping with our previously established hypothesis that YK11 behaves similarly to DHT-derived AAS, the following side may effects may present themselves while taking YK11:
Suppression of Natural Testosterone
This occurs with all AAS and SARMs, so it’s most likely the case that YK11 is no different in this regard. Following through with our DHT-derived AAS hypothesis, there is clear evidence that the DHT-derived AAS stanozolol causes suppression of natural testosterone levels in men [
9].
Male-pattern Baldness (Androgenic Alopecia)
Androgenic alopecia, which is balding that occurs at the vertex in men, has been shown to be caused primarily by DHT [
10].
Liver Toxicity (Hepatotoxicity)
Orally ingested 17α(alpha)-alkylated AAS such as oxandrolone (Anavar™) and methandienone (Dianabol™) are notorious for causing toxicity of the liver due to their structural modification that allows them to clear the first pass of the liver. However, it’s not known if YK11 would cause such an effect. While YK11 is orally ingested like other SARMs, it still maintains a greater amount of selectivity compared to AAS in general, which should hypothetically make cases of liver toxicity less likely to occur. Plus, they’re not chemically modified in the same way that 17α(alpha)-alkylated AAS are.
But anecdotal reports have shown increases in liver enzymes while taking YK11. Why this occurs remains unknown. But it could be speculated that users who reported these elevated liver enzymes may have been administering other compounds alongside YK11 that made liver toxicity more likely to occur. But of course we won’t know for certain until researchers narrow down a potential mechanism that lies behind YK11’s potential to cause liver toxicity.
YK11 vs. Other SARMs
Taking into consideration that YK11’s mechanism of action differs so widely from other SARMs in addition to the fact that it’s severely lacking in both animal and human data compared to other SARMs, there wouldn’t be any value in drawing any bold conclusions regarding how YK11 compares with other SARMs. The information/data just isn’t there.
All that can really be said is that it’s probably best to use YK11 in a supplementary role as an addition to a performance-enhancing stack, rather than using it in isolation. In this way, you can really take advantage of YK11’s unique follistatin-expression mechanism in conjunction with other performance-enhancing compounds.
Dosage
It should be made clear that there is no “standard” dose for recreational use, and that any dosage amounts you may see across the internet are derived anecdotally. With that being said, it appears that anywhere from 10-30mg per day is relatively common, with the lower end of the range being significantly more common than the higher end of the range. Therefore, it’s probably not necessary to wander above 20mg to observe notable results.
Establishing a dosing protocol is also difficult since there is still no data available on YK11’s bioavailability and half-life. On the bright side, we do have data from research that was conducted in order to establish standardization for drug testing, such as those looking to illicitly use SARMs like YK11 in competitive organizations that explicitly prohibit their use. While all of YK11’s metabolites are not fully known, it has been reported that metabolites are still detectable in excreted urine 36-48 hours after administration [
11].
While this doesn’t tell us the half-life of YK11, this does allow us to speculate that its half-life may be similar to other SARMs like LGD-4033, ranging from 24-36 hours [
12]. So it’d be wise to take it once per day at the very least, with 2x/day dosing most likely being your best bet so that you can ensure you have an equal blood concentration of YK11 throughout the day rather than dramatic rises and falls.
Should I Use a Post-Cycle Therapy (PCT) After My YK11 Cycle?
It’s highly recommended that if you cycled with YK11, you should complete a PCT after you discontinue its use. This is especially true if you took it alongside other AAS or SARMs. While it hasn’t been shown in human trials as of this publication, one risks suffering from a low testosterone condition if one does not undergo a PCT after discontinuation of use.
Provided our previous postulation that YK11 most likely has a half-life in the range of 24-36 hours, one should start their PCT the day after they stop taking YK11.
It’s not necessary to include aromatase inhibitors in your PCT. Using a selective estrogen receptor modulator (SERM) should suffice; the two most popular choices being either tamoxifen (Nolvadex®) or clomiphene (Clomid®). While using tamoxifen (Nolvadex®) is recommended due to its milder nature, some prefer the use of clomiphene (Clomid®). Either way, one or the other should be just fine. Rarely is it necessary to take both of these SERMs simultaneously in order to recover properly.
No matter which one you choose, here’s what a simple 4-week PCT protocol would look like for these SERMS:
Week |
Tamoxifen (Nolvadex®) |
OR |
Clomiphene (Clomid®) |
1 |
40 mg/day |
50 mg/day |
2 |
40 mg/day |
50 mg/day |
3 |
20 mg/day |
25 mg/day |
4 |
20 mg/day |
25 mg/day |
References
- Lee S.J., Gharbi A., Shin J.E., Jung I.D., Park Y., (2021). Myostatin inhibitor YK11 as a preventative health supplement for bacterial sepsis. Biochemical and Biophysical Research Communications. 543, 1-7
- Matsumoto, T., Shiina, H., Kawano, H., Sato, T., & Kato, S. (2008). Androgen receptor functions in male and female physiology. The Journal of steroid biochemistry and molecular biology, 109(3-5), 236–241. https://doi.org/10.1016/j.jsbmb.2008.03.023
- Lai, J. J., Chang, P., Lai, K. P., Chen, L., & Chang, C. (2012). The role of androgen and androgen receptor in skin-related disorders. Archives of dermatological research, 304(7), 499–510. https://doi.org/10.1007/s00403-012-1265-x
- Rodriguez, J., Vernus, B., Chelh, I., Cassar-Malek, I., Gabillard, J. C., Hadj Sassi, A., Seiliez, I., Picard, B., & Bonnieu, A. (2014). Myostatin and the skeletal muscle atrophy and hypertrophy signaling pathways. Cellular and molecular life sciences : CMLS, 71(22), 4361–4371. https://doi.org/10.1007/s00018-014-1689-x
- McPherron, A. C., Lawler, A. M., & Lee, S. J. (1997). Regulation of skeletal muscle mass in mice by a new TGF-beta superfamily member. Nature, 387(6628), 83–90. https://doi.org/10.1038/387083a0
- McPherron, A. C., & Lee, S. J. (1997). Double muscling in cattle due to mutations in the myostatin gene. Proceedings of the National Academy of Sciences of the United States of America, 94(23), 12457–12461. https://doi.org/10.1073/pnas.94.23.12457
- Kambadur, R., Sharma, M., Smith, T. P., & Bass, J. J. (1997). Mutations in myostatin (GDF8) in double-muscled Belgian Blue and Piedmontese cattle. Genome research, 7(9), 910–916. https://doi.org/10.1101/gr.7.9.910
- Matzuk, M. M., Lu, N., Vogel, H., Sellheyer, K., Roop, D. R., & Bradley, A. (1995). Multiple defects and perinatal death in mice deficient in follistatin. Nature, 374(6520), 360–363. https://doi.org/10.1038/374360a0
- Small, M., Beastall, G. H., Semple, C. G., Cowan, R. A., & Forbes, C. D. (1984). Alteration of hormone levels in normal males given the anabolic steroid stanozolol. Clinical endocrinology, 21(1), 49–55. https://doi.org/10.1111/j.1365-2265.1984.tb00135.x
- Piraccini, B. M., & Alessandrini, A. (2014). Androgenetic alopecia. Giornale italiano di dermatologia e venereologia : organo ufficiale, Societa italiana di dermatologia e sifilografia, 149(1), 15–24.
- Piper, T., Dib, J., Putz, M., Fusshöller, G., Pop, V., Lagojda, A., Kuehne, D., Geyer, H., Schänzer, W., & Thevis, M. (2018). Studies on the in vivo metabolism of the SARM YK11: Identification and characterization of metabolites potentially useful for doping controls. Drug testing and analysis, 10(11-12), 1646–1656. https://doi.org/10.1002/dta.2527
- Basaria, S., Collins, L., Dillon, E. L., Orwoll, K., Storer, T. W., Miciek, R., Ulloor, J., Zhang, A., Eder, R., Zientek, H., Gordon, G., Kazmi, S., Sheffield-Moore, M., & Bhasin, S. (2013). The safety, pharmacokinetics, and effects of LGD-4033, a novel nonsteroidal oral, selective androgen receptor modulator, in healthy young men. The journals of gerontology. Series A, Biological sciences and medical sciences, 68(1), 87–95. https://doi.org/10.1093/gerona/gls078